Tips for choosing the optimal preclinical model: focus on atherosclerosis and LDLR
Cardiovascular diseases (CVDs) are the leading cause of morbidity and mortality worldwide.1 The majority of CVDs result from atherosclerosis, a chronic disease where the flow of oxygen-rich blood to organs is strongly limited by the formation and accumulation of fibrofatty lesions in the artery wall. This can ultimately lead to myocardial infarctions, strokes, and disabling peripheral artery diseases.2 Several experimental and epidemiological evidences have shown that disturbances in lipoprotein metabolism play a crucial role in atherosclerosis: for example, low-density lipoprotein (LDL) increases the risks of atherosclerosis and coronary heart disease, while high-density lipoprotein (HDL) acts the opposite way.3–6
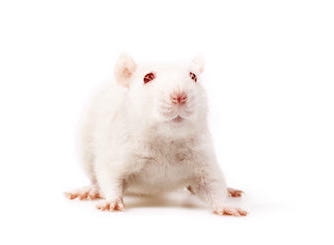
With the advances in genetic engineering, mice and rats have now become the chosen model species to investigate atherosclerosis, but they still present limiting characteristics. Indeed, while rodents transport most of their cholesterol in HDL particles, humans move cholesterol in LDLs.7 Consequently, mice and rats overall have massively lower cholesterol levels, do not develop atherosclerosis, and require genetic, dietary and/or mechanical modifications to be used as disease models.8
One example of genetic modification used as a disease model in atherosclerosis studies is the mutation of the LDL receptor (LDLR). This transmembrane glycoprotein mediates the clearance of circulating LDLs, and is involved in controlling the amount of cholesterol in the blood by mediating LDL endocytosis.9,10 In humans, loss-of-function mutations in LDLR are associated with familial hypercholesterolemia (FH), an autosomal dominant genetic disorder characterized by a massive elevation of total plasma cholesterol, premature atherosclerotic CVDs, and early death.3,11Unfortunately, Ldlr Knockout (KO) mice show a moderate increase in LDL-cholesterol levels and develop atherosclerotic lesions only if fed a high-cholesterol diet (and even then, the rate, site, and the nature of such lesions differ from humans).12
Interestingly, rats possess a lipid metabolism with characteristics in between those of humans and mice. In addition, rats differ from mice in their inflammatory responses and cholesterol metabolism. As a result, Ldlr KO rats display high levels of LDL cholesterol, increased adiposity, and progressive glucose intolerance more closely resembling what is observed in humans than Ldlr KO mice. However, Ldlr KO rats have been generated and characterized only recently – the first Ldlr KO mouse was developed 10 years earlier – and thorough comparative studies between Ldlr KO rats and FH patients are still lacking.13
With the advent of CRISPR/Cas9, targeted gene deletion in rats has become easier and more common, allowing scientists to establish rat models to try and overcome certain limitations of mice, especially when studying disorders such as atherosclerosis. In 2018, researchers generated rats that were invalidated for both Ldlr and Apoe, another protein that participates in the transport of cholesterol-rich lipoproteins, and showed that Apoe/Ldlr double-KO rats display sex difference profiles in atherosclerotic plaque formation very similar to humans.14
Further studies on these models will certainly help scientists to gain a better understanding of atherosclerosis physiopathology, and promote additional therapeutic studies. Moreover, thanks to gene editing tools such as CRISPR/Cas9, more sophisticated rat models will be developed with better translatability to humans, hopefully benefiting future development of more powerful therapeutics for this widespread disease.
References:
- Cardiovascular diseases (CVDs). https://www.who.int/news-room/fact-sheets/detail/cardiovascular-diseases-(cvds) (2021).
- Libby, P. et al. Atherosclerosis. Nat Rev Dis Primers 5, 56 (2019).
- Wang, H. Y. et al. A lipidomics study reveals hepatic lipid signatures associating with deficiency of the LDL receptor in a rat model. Biology Open 5, 979–986 (2016).
- Brunham, L. R. & Hayden, M. R. Human genetics of HDL: Insight into particle metabolism and function. Progress in Lipid Research 58, 14–25 (2015).
- Ishibashi, S., Goldstein, J. L., Brown, M. S., Herz, J. & Burns, D. K. Massive xanthomatosis and atherosclerosis in cholesterol-fed low density lipoprotein receptor-negative mice. J. Clin. Invest. 93, 1885–1893 (1994).
- Zhong, S. et al. An update on lipid oxidation and inflammation in cardiovascular diseases. Free Radical Biology and Medicine 144, 266–278 (2019).
- Sari, G. et al. A mouse model of humanized liver shows a human-like lipid profile, but does not form atherosclerotic plaque after western type diet. Biochemical and Biophysical Research Communications 524, 510–515 (2020).
- Oppi, S., Lüscher, T. F. & Stein, S. Mouse Models for Atherosclerosis Research—Which Is My Line? Front. Cardiovasc. Med. 6, 46 (2019).
- Brown, M. S. & Goldstein, J. L. A Receptor-Mediated Pathway for Cholesterol Homeostasis. Science 232, 34–47 (1986).
- Ishibashi, S. et al. Hypercholesterolemia in low density lipoprotein receptor knockout mice and its reversal by adenovirus-mediated gene delivery. J. Clin. Invest. 92, 883–893 (1993).
- Kim, Y. R. & Han, K. H. Familial Hypercholesterolemia and the Atherosclerotic Disease. Korean Circ J 43, 363 (2013).
- Sithu, S. D. et al. Atherogenesis and metabolic dysregulation in LDL receptor–knockout rats. JCI Insight 2, e86442 (2017).
- He, K. et al. An interspecies study of lipid profiles and atherosclerosis in familial hypercholesterolemia animal models with low-density lipoprotein receptor deficiency. Am J Transl Res 11, 3116–3127 (2019).
- Zhao, Y. et al. Hyperlipidemia induces typical atherosclerosis development in Ldlr and Apoe deficient rats. Atherosclerosis 271, 26–35 (2018).
Related products
Catalogue product
Customized product
Scientific excellence
From model design to experimental results
Tailor-made solutions adapted to scientific questionsRobust validation data on catalog models
Comprehensive dataset package
Generated with biopharma partners and in-houseCustomer care
Scientific follow-up and advice along the project
Collaborative approach for problem solving and development of innovative modelsEasy and fast access to models
Breeding facilities in US and Europe
Certified health status from professional breeders