iPS technology: Four factors that will change the world… some day
A decade ago, one paper struck like thunder an entire domain of research, and a pretty booming and competitive one at that: stem cell biology. Researchers from Kyoto University showed that by simply overexpressing the right combination of factors, an adult differentiated cell could acquire pluripotency.(1)
Pluripotent stem (PS) cells have the ability to self-renew endlessly, and to differentiate into a plethora of cell types. Before 2006, they were directly isolated from embryos, or obtained by reprogramming unfertilized eggs using a rather complex and tedious method. Needless to say, neither of these options is practical, the former raising major ethical issues when working with human embryos. In addition, working on pluripotency in human cells is very demanding, not to say a bit of a pain. Aside from being subject to numerous regulations, PS cells need rigorous and continuous care. Then why bother keeping using these cells? Well, if you have cells that can generate different tissues in a body, not only is it of great interest in knowing how these tissues form but it also provides wonderful insight into how they regenerate. And with a worldwide population that is rapidly aging, regeneration and regenerative medicine concentrate a lot of interests.
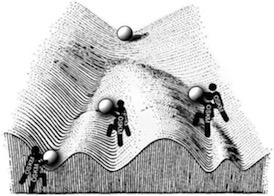
‍
So, when Shinya Yamanaka and colleagues showed that PS cells could be obtained by introducing four genes in an adult cell, it was indeed a revolution. They first hypothesized that an adult differentiated cell, from the skin in that case, could acquire pluripotency by simply expressing the right combination of transcription factors. They started with 24 and narrowed it down to just four factors: Oct4, Sox2, Klf4, and cMyc,(2) now known as OSKM or Yamanaka factors. They called these reprogrammed cells induced PS (iPS) cells, and the following year showed they could do the same from a human skin cell.(3) Providing a virtually inexhaustible source of human PS cells, iPS technology rapidly flowed to pretty much every research institute in the world.
At the beginning, this was identified as a major medical breakthrough that could transform cell therapy. The idea was to isolate easily accessible differentiated cells from a patient, make iPS cells from them, “correct” the genetic defect causing the disease, differentiate them into the cell type that shows the phenotypic alteration (blood cells, retina cells...), and reintroduce them in the patient to repopulate the failing organ. Some 10 years later, however, only one clinical trial is ongoing, to treat macular degeneration. After a first successful transplantation of retinal pigment epithelium (RPE) sheets obtained through the reprogramming and differentiation of a patient’s own cells in 2014,(4) the trial was put on hold one year later, after genetic alterations were found in a second patient’s iPS cells. It resumed earlier this year with a patient receiving for the first time reprogrammed cells from a donor,(5) but for now iPS cells have revolutionized the bench more than the bedside.
Although they haven’t yet fulfilled their promises in therapeutics, iPS cells are now routinely used in an arm-long list of disciplines, including developmental and regenerative biology, neuroscience, cardiology, hepatology, disease-modeling, and even drug discovery. This technology has allowed major advances in the elucidation of pathological mechanisms of numerous diseases such as Alzheimer’s and Parkinson’s.
Many efforts have been concentrated toward differentiating human iPS cells obtained from healthy donors or patients into specialized cell types, including hepatocytes or cardiomyocytes to test new drugs’ toxicity. This might even become the norm in drug safety assessment one day, with the CiPA (Comprehensive in vitro Proarrhythmia Assay) initiative’s new paradigm.(6) This organization, regrouping researchers, medical agencies, and nonprofit institutions from all over the world (USA, Europe, Japan, Canada, etc.), and focusing on setting optimized rules to test new molecules’ potential cardiotoxicity, recently suggested to include in vitro assays in hiPS-derived cardiomyocytes.(7) But—and unfortunately there is a but—most of these iPS-derived cells present immature characteristics, resembling more fetal than adult differentiated cells, calling for optimized protocols and techniques.
In addition to these limitations, it is fascinating to think that we still don’t entirely understand reprogramming. For example, we can dissect the process in successive steps and we can validate the pluripotency of the reprogrammed cells many different ways, but we still don’t know exactly how it works, how four factors can induce such a drastic change of fate. With these questions, and some others still unsolved (the risk of tumorigenicity is a real obstacle), major breakthroughs most likely still lie ahead of us with iPS technology.
References:
- Takahashi, K. & Yamanaka, S. Induction of Pluripotent Stem Cells from Mouse Embryonic and Adult Fibroblast Cultures by Defined Factors. Cell 126, 663–676 (2006).
- Ibid.
- Takahashi, K. et al. Induction of Pluripotent Stem Cells from Adult Human Fibroblasts by Defined Factors. Cell 107, 861–872 (2007).
- Mandai, M. et al. Autologous Induced Stem-Cell–Derived Retinal Cells for Macular Degeneration. N. Engl. J. Med. 376, 1038–1046 (2017).
- Japanese man is first to receive 'reprogrammed' stem cells from another person.
- Gintant, G., Fermini, B., Stockbridge, N. & Strauss, D. The Evolving Roles of Human iPSC-Derived Cardiomyocytes in Drug Safety and Discovery. Cell Stem Cell 21, 14–17 (2017).
- CIPA
Ultimate predictive models
Physiological relevance
Comprehensive and reliable validation data
Over 600 scientific articles based on our modelsScientific excellence
In-depth expertise in preclinical science, physiology, and model development
Unique R&D platform located in FranceCustomer-centric
Tailor-made approach to your needs
Strong emphasis on customer satisfactionCollaborative partner
Long-standing partnerships with 17 of the top 20 pharma companies
Co-development of innovative models for next-generation drugs
Guaranteed FTO/freedom to operate